The hunt for new physics in the dark sector
The standard model of particle physics, which describes the nature and interaction of sub-atomic particles, is one of the most celebrated theories in science. Over the years, its predictions have been verified to incredible precision and few discrepancies have persisted. But despite its many successes, we know the model is incomplete, among other things it fails to explain the presence of the dark sector – such as the mysterious dark matter and dark energy, which are the main components of the standard cosmological model, and massive neutrinos. Direct measurements of dark matter and dark energy remain out of reach, their ‘dark’ nature means they hardly interact, making them extremely difficult to detect through particle physics experiments. Their presence has only been detected in astronomy through their effect on galaxies and the distribution of matter on the very largest scales. Experiments in cosmology have shown us that we need dark matter, specifically cold dark matter, to explain observations of galaxy rotation curves, the cosmic microwave background, and to provide the seeds for the growth of structures (and eventually galaxies like our own Milky Way). On the other hand, Dark energy, in the form of a cosmological constant, provides an explanation for the accelerated expansion of the Universe at late times.
What can we expect to learn about the dark sector from the next generation of cosmological experiments?
Dark Matter is an essential component of the standard model of cosmology, without it we cannot explain how galaxies form and how the universe looks as it does today. Despite its necessity, we really have no real idea about what it really is. There is plenty of room for new and exotic physics here, which for me makes it the most intriguing component of the dark sector. Is dark matter a single particle, or many? Is dark matter interactive or is it boring (as assumed in the standard model)? The absence of any direct measurements suggests dark matter interactions with other particles and forces other than gravity must be very weak. Assuming this is the case, then we are left with dark matter models that only influence things, distinctively, through their mass. In the standard model, dark matter is assumed to be cold, meaning massive and slowly moving. This allows dark matter to form clumps on small scales, since the dark matter particles can efficiently coalesce. But this need not be the case, warm dark matter, formed of slightly lighter particles and moving at greater speeds will not be able to form very small clumps. By measuring the number of small clumps in the universe, we can begin to understand how heavy dark matter particles really are. There are of course more exotic models, take for example fuzzy dark matter, made of very light particles, so light that they begin to exhibit quantum mechanical effects on galactic scales – here they will produce distinctive quantum interference patterns. Unfortunately, at small scales it becomes rather difficult to disentangle the effects of dark matter from baryonic feedback, a term collectively describing the effect of supernovae, black holes, gas, etc. This is a major obstacle, and one which only sophisticated simulations with carefully calibrated galaxy formation models can help to distinguish.
All of this assumes dark matter is rather boring, and while the evidence suggests dark matter interacts very weakly with known particles and forces, we have little evidence to suggest whether dark matter interacts with other components of the dark sector – whether that be with itself (if dark matter is not a single particle) or with dark energy. The latter is an extremely interesting proposition, as it can resolve several discrepancies seen in cosmology today – such as the Hubble tension and S8 tension. These describe discrepancies between measurements of the expansion rate and “clumpy-ness” from early and late universe measurements. Furthermore, It would be able to explain a seemingly unrelated anomaly. For some time, we have found that voids produce larger than expected imprints on the very earliest light in the universe, the cosmic microwave background. So far, this excess has been a puzzle but interactions between the dark sector can explain this signal. Measuring this signal more precisely will enable us to make a unique test for interactions in the dark sector. This would have a profound impact on our understanding of fundamental physics and the dark sector, particularly as the latter would imply dark energy is not a cosmological constant (more on this later).
Dark Energy. Since the early 20th century, and the work of Edwin Hubble, we have known the universe is expanding. This empirical discovery is what led cosmologists away from the more prevalent static universe theories of the past to models where the universe evolves over time. If we run the clock backwards, we get to a stage where the universe is infinitely hot and dense – the big bang. By the early 90s, theories of the big bang were well established, and cosmologist began to try to measure how much the universe’s expansion rate was slowing down. A universe full of matter, as was believed at the time, would resists expansion due to the forces of gravity, but to their great surprise the universe’s expansion rate was accelerating. Although there had been signs of dark energy before, this measurement was the definitive proof. Dark energy is used generally to describe a component of the universe responsible for driving the accelerated expansion. In the standard model we assume dark energy is a cosmological constant, meaning It is represented by a single constant value over all of space time. While a cosmological constant is rather easy to explain in general relativity (Einstein’s theory of gravity), its small value is very difficult to reconcile with fundamental physics. This has led many to wonder whether dark energy is something else. The next generation of cosmological experiments will test for deviations from the cosmological constant, by exploring whether dark energy evolves over time or whether dark energy is really a symptom of an incorrect theory of gravity. For the moment, the cosmological constant appears to be standing strong, but we may look back to the discrepancies and anomalies present is cosmology as early signs that dark energy is really something else.
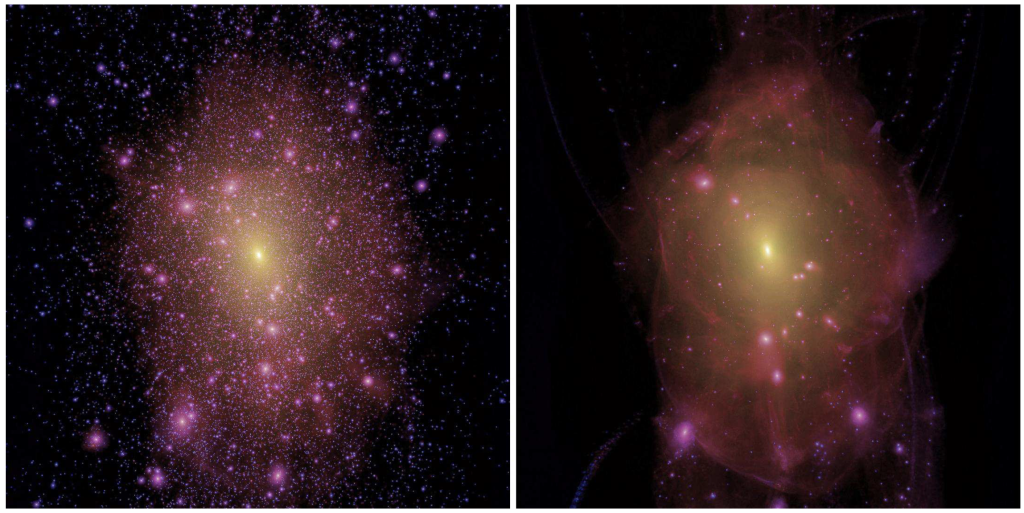
A comparison of haloes (clumps of matter) for different dark matter models. On the left is a halo in a model with cold dark matter and on the right is a model with warm dark matter. Cold dark matter produces more small clumps than warm dark matter. Figure from Lovell et al. (2012), MNRAS, 420, 3, 2318–2324, https://doi.org/10.1111/j.1365-2966.2011.20200.x
Neutrinos are a rather unique component of the dark sector. They are chargeless cousins to the sub-atomic electron particle, and until the late 90s (and the discovery of neutrino oscillations) were thought to be massless. Their elusive nature has made determining their exact mass difficult, with particle physics only able to provide lower limits. Cosmology has so far only been able to provide upper bounds, but these upper bounds are slowly approaching particle physics lower bounds, meaning a measurement should be made in the near future. Massive neutrinos influence the distribution of matter by preventing structures at small scales from forming, an effect caused by their high thermal velocities.
The one concern with this detection, when/if it is made, is that it will be dependent on a cosmological model. But what if this model is wrong? Current tensions in cosmology suggest there may be issues with the assumptions of the standard model of cosmology, so what do we do if these persists? Are neutrino mass determinations destined to come with a caveat? It appears, at least for the immediate future, that this may be the case. This of course assumes we are confined to standard techniques. Another approach is to look for unique neutrino effects on other cosmological observables – such as its effects on the cosmic web, and in particular filaments, which could prove more accurate probes of its mass and provide additional consistency checks for the assumed cosmological model.
So, what is lurking in the dark? Is dark matter interactive? Is dark energy changing over time or is it a symptom of an incorrect theory of gravity? Will we measure neutrino mass reliably? Over the next few years large galaxy surveys (such as DESI, Euclid and LSST) will shed further light on the dark sector. Will they reveal new physics – or will the simple assumptions of the resilient standard model of cosmology triumph again? Only time will tell.